Chapter 7 Microbial Social Networks
The objectives of this topic are to:
- Realize that bacteria interact with one another and with higher organisms in ways similar to how higher organisms react.
- Realize that bacteria can communicate via chemistry to mediate interactions.
- Realize that bacterial interactions with higher organisms are important.
Figure 7.1: Types of Microbial Interactions
7.1 Symbiosis
This is an association of two or more diferent species of organisms (something coined by H.A. deBary in 1879).
Furthermore, note the following terms for microbial interactions:
- Ectosymbiont: an organism located on the surface of another organism.
- Endosymbiont: an organism located within another organism.
- Symbiont: refers to physical contact between dissimilar organisms of similar size.
7.2 Mutualism
This is a type of symbiosis that results in some benefit to both partners. A mutualistic relationship also has some degree of obligation: both partners are unable to live separately (i.e, both the mutualist and the host depend on each other).
For instance, endosymbiotic microbes provide much-needed vitamins and amino acids, aphid-Buchnera aphidicola interactions, insect-Wolbachia interactions, deep sea tube worms-bacteria, and coral-Zooxanthellae relationships:
Figure 7.2: Coral-Zooxanthellae Relationships
Figure 7.3: Deep Sea Tube Worms and Bacterial Relationships
7.3 Cooperation
This is a positive, but not obligate form of symbiosis which involves syntrophic relationships.
While both organisms in cooperation benefit, this is unlike mutualism as it is not obligatory.
Relationships between sulfide-oxidation bacteria and a variety of animals are examples of cooperation (e.g., shrimp and sulfide-oxidation bacteria).
7.4 Commensalism
Here, one organism benefits (the commensal) and the other is unaffected.
The relationship here is often syntrophic and may also involve the modification of one environment by one organism (hence making it more suitable for another organism).
Nitrification by nitrifying bacteria are an example of commensalism:
Figure 7.4: Nitrification
More examples of commensalism include:
- Microbial succession during the spoilage of milk.
- Formation of biofilms.
- Surface microbes on plants or animals
7.5 Predation
This type of symbiosis involves a predator species that attacks and (usually) kills its prey.
Bdellivibrio bacteria penetrates cell walls and grow outside the plasma membrane.
Vampirococcus bacteria has an epibiotic mode (i.e., these bacteria secrete enzymes that digest their prey from the inside out) of attacking prey.
Daptobacter bacteria penetrates its prey before directly consuming their cytoplasmic contents.
Myxococcus bacteria form a “wolf pack”; cells use gliding motility to creep, overtake their prey, and release degradative enzymes:
Figure 7.5: M. xanthus Predation
Predation may produce a microbial loop (organic matter made by autotrophs are mineralized); microbial predators may also provide nutrients for primary producers.
7.6 Parasitism
In this relationship, one organism benefits (the parasite) and the other is harmed (the host).
There is always some degree of co-existence between the host and the parasite: successful parasites have evolved to co-exist in equilibrium with their hosts (upsetting of which might cause the host or the parasite to die).
Rickettsia typhi is the causative agent of Typhus: it is harbored on fleas, lives on rats, and is transmitted to humans via flea bites. Typhus is endemic within a population until societal changes (e.g., war) occur and cause the disease to become an epidemic.
A consequence of parasitism is genomic reduction. The parasite may lose unused genomic information as a result of long-term parasitism.
7.7 Ammensalism
Here, there is a negative effect on one organism due to the release of a specific compound.
Some specific examples include:
- Antibiotic production by fungi and bacteria
- Bacteriocin production by bacteria
- Production of antibacterial peptides by insects and animals (e.g., defensins, cecropins, and athelicidins)
- Production of organic acids during fermentation
- Ants utilizing antibiotic-producing streptomycin to control fungal parasites
7.8 Competition
This occurs when two organisms try to acquire or use the same resource. Because of this, there are two possible outcomes:
- One organism dominates
- Two organisms overlap too much in their resource usage, so one population dies out the other one prevails.
- Two organisms share the same resource
- However, both organisms survive at lower population numbers.
7.8.1 Ant fungus farming
Leaf cutting ants grow fungi as food, yet fungus gardens are susceptible to invasion by virulent fungal strains.
Luckily, this invasion is prevented by antibiotic-producing bacteria symbionts.
Figure 7.6: Relationship Between Leaf Cutting Ants and Antibiotic-Producing Bacteria
7.9 Means of Communication in the Microbe World
Bacteria communicate via density-dependent chemical communications (hence leading to a rapid induction of phenotypes). Gene expression coordination at the population level is a widespread phenomenon among bacteria.
Hence, these “chemical communications” modulate phenotypes that modulates:
- Virulence
- Asociation with higher organisms or surfaces
7.9.1 Discovery of cell-cell communication by bacteria
Nealson et al. (1970) showed that luminescence in Vibrio fischeri is controlled by a self-produced chemical signal called the autoinducer.
Eberhard et al. (1981) identified the autoinducer signal as homoserine lactone.
Engebrecht et al. (1983) then cloned genes for the autoinducer signal, receptor, and the lux genes.
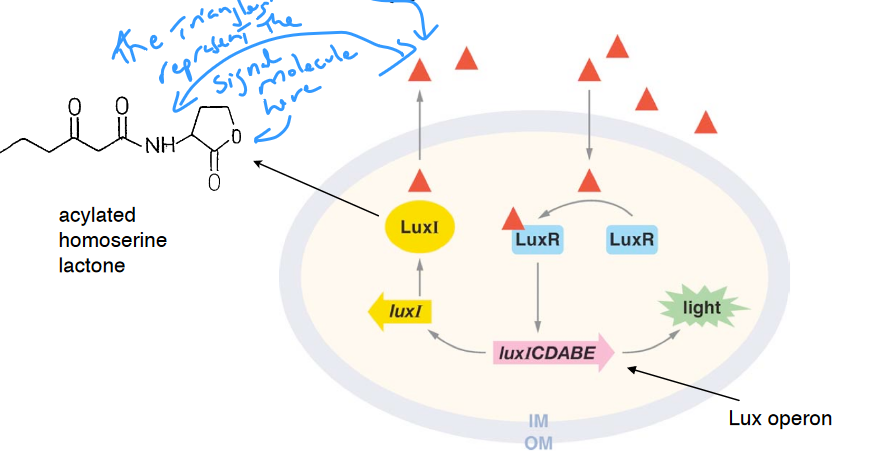
Figure 7.7: Mechanism for Luminescence in Vibrio fischeri
NB: the blue text in the picture states - “[t]he triangles represent the signal molecule here.”
Nevertheless, the autoinducer accumulates outside the cell and is recognized by a specific receptor.
Thereafter, a group response is generated once a critical threshold concentration is reached. Cellular responses extend beyond the physiological changes needed to metabolize or detoxify the autoinducer.
Some other examples include:
Figure 7.8: Other Forms of Density-Dependent Communications
7.9.2 Another example
Furanones are shown to stop AHL-mediated infections and bleaching by epiphytic bacteria in corals.
Coral bleaching is observed in the late summer (where ocean temperatures reach 24 degrees Celsius) and occurs at the mid-thallus where furanone concentrations are lower.
Furthermore, bleaching has also been found in temperature regulated in V. shiloi infection of O. patagonica (a coral species).