Chapter 4 Krebs’ Cycle (i.e., the TCA cycle)
When energy from ATP is utilized in cellular reponses (e.g., muscle contraction), ATP becomes cleaved to form ADP and Pi. In cellular respiration, O2 is used to generate ATP from the oxidation of fuels to CO2.
Figure 4.1: Two Phases of Respiration
In phase 1 (see above) of cellular respiration from glucose, electrons are transferred to the coenzymes NAD+ and FAD and consequently reduced to NADH and FADH2.
It is worth noting that the oxidation of most fuels always generate a two carbon acetyl group (i.e., acetyl CoA). The TCA cycle is where the oxidation of the acetyl group occurs - energy here is mostly collected as NADH and FADH2.
4.1 A Quick Recap of Chapter 3
4.1.1 Why is ATP a “high energy compound?”
ATP has at least one bond with a favorable \(\Delta G^\omicron\) during hydrolysis. It does not mean that the compound is highly unstable or highly reactive!
ATP is kinetically stable and its breakdown is coupled with a thermodynamically unfavorable reaction, like the phosphorylation of glucose to form glucose-6-phosphate:
Figure 4.2: Glucose 6 Phosphate Formation
It is more appropriate to say that ATP has a high phosphoryl group transfer potential!
4.1.2 Oxidation
Carbon atoms are oxidized when they lose a hydride ion (i.e., H-) or are combined with oxygen. Dehydrogenases are enzymes that catalyze the removal of hydrogen from a carbon atom:
Figure 4.3: Accepting and Donating Hydride Ions
4.1.3 Converting pyruvate into ethanol
Yeast cells convert pyruvate into ethanol and CO2 gas via the oxidation of NADH to NAD:
Figure 4.4: Alcohol Generation from Pyruvate
Do revisit the previous chapter if you need a refresher on this!
4.2 Substrate-Level Phosphorylation and Concepts
4.2.1 Pyruvate Dehydrogenase Complex (i.e., PDC)
Figure 4.5: Reaction Catalyzed by PDC
The PDC is a cluster of three different enzymes:
- Pyruvate dehydrogenase (E1)
- Dihydrolipoyl transacetylase (E2)
- Dihydrolipoyl dehydrogenase (E3)
A total of five coenzymes are required in this complex: TPP, coenzyme A, lipoic acid, FAD, and NAD+.
This reaction happens in the mitochondrial matrix in eukaryotic cells!
4.2.1.1 Organization of the E. coli PDC
Figure 4.6: Structure of the PDC
E2 (24 subunits total) forms trimers that associate with the corners of the cube to form a particle with cubic symmetry.
E1 (24 subunits) forms dimers that associate with the core of the cube; E3 (12 subunits) forms dimers that associate with the center of the cube’s six faces.
The PDC is hence a 60-subunit cube of E1, E2, and E3 proteins.
4.2.2 Acetyl-coenzyme A (i.e., Acetyl-CoA)
Figure 4.7: Structure of Acetyl-CoA
Acetyl-CoA serves to transfer acetyl groups to molecules; its reactive portion is a thiol (i.e., the -SH portion).
A high-energy thioester (\(\Delta G^\omicron = -31.5 \text{ kJ / mol}\)) is also present in this molecule. A large amount of free energy is released upon the hydrolysis of the thioester - this is why Acetyl-CoA has a high acyl group transfer potential.
CoA is a common product of carbohydrate, fatty acid, and amino acid breakdown.
4.2.2.1 Resonance energies
Figure 4.8: Free Energy from Hydrolysis of Thioesters and Oxygen Esters
The high \(\Delta G^\omicron\) is due to the lack of resonance stabilization. Most esters have two resonance forms, and the stabilization of the resonance form involves the overlapping \(\pi\)-electron orbital overlap (hence giving partial double bond properties).
It is because of this lack of partial double bond character that the S-R bond of acetyl-CoA is a good leaving group in nucleophilic displacement reactions. Hence, acyl groups are readily transferred over to other molecules.
4.2.3 Interconversion of lipoamide and dihydrolipoamide
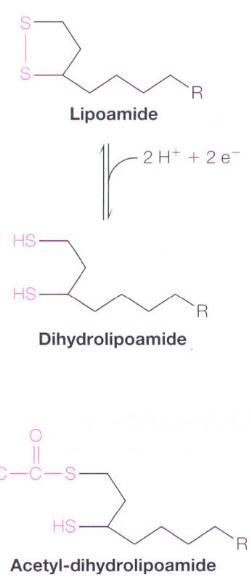
Figure 4.9: Lipoamide and Dihydrolipoamide
A lipoamide molecule is a lipoic acid molecule linked to the \(\epsilon\)-amino group of a Lys residue via an amide linkage. Dihydrolipoamide is the product of reducing the disulfide to a dithiol.
4.2.4 Tyrosine Pyrophosphate (i.e. TPP)
Figure 4.10: Structure of TPP
This is a pyrophosphate of vitamin B1 whose function is to transfer an activated aldehyde unit.
The functional group of this pyrophosphate is the thiazolium ring (which has an acidic proton at C2). The loss of that proton produces a carbanion: the active species - this loss is catalyzed by E1 of the pyruvate dehydrogenase complex.
4.2.4.1 TPP in the pyruvate dehydrogenase reaction
Figure 4.11: TPP in the Pyruvate Dehydrogenase Reaction
First (1), there is a nucleophilic attack by the ylid form of TPP on the carbonyl carbon of pyruvate. This causes CO2 to be evolved and hydroxyethyl TPP to be formed (2).
This (3) is followed by the transfer of the hydroxyethyl group to the lipoamide moiety via an attack of the hydroxyethyl carbanion on the lipoamide disulfide, followed by the elimination of TPP to form an acetyl thioester on dihydrolipoamide; E1 is also regenerated here.
Thereafter (4), the acetyl group is transferred over to CoA. This is a nucleophilic acyl substitution reaction that exchanges one thioester for another - this yields acetyl-CoA and dihydrolipoamide.
The last two steps (5) are needed to reoxidize the dihydrolipoamide of E2 and transfer the pair of electrons via FAD to NAD+.
4.2.5 TPP deficiency
A lack of vitamin B1 leads to a TPP deficiency that inhibits enzymes from requiring TPP as a coenzyme.
Consequently, E1 of the complex is inhibited and the brain suffers as a result. A disease known as beriberi (coined by Jacob Bonitus in 1630) can occur as a result, leading to neurological and cardiovascular disorders.
A thiamine deficiency can be detected as increased pyruvate levels and low enzyme activity (especially of transketolase) in the blood. This be can reversed by consuming vitamin B1.
4.3 The TCA Cycle
The TCA cycle is a way of cleaving two-carbon compounds. In many instances, the cleavage happens between the \(\alpha\) and the \(\beta\) carbons; in the case of \(\alpha\)-hydroxyketone, the cleavage happens between the carboxyl carbon and the \(\alpha\) carbon.
However, none of the strategies mentioned above are suitable for cleaving acetate (it has no \(\beta\) carbons and the second method would involve hydroxylation). Hence, acetate is first condensed with oxalatoacetate - CO2 is formed, oxalatoacetate is regenerated, and metabolic energy from NADH and ATP is captured.
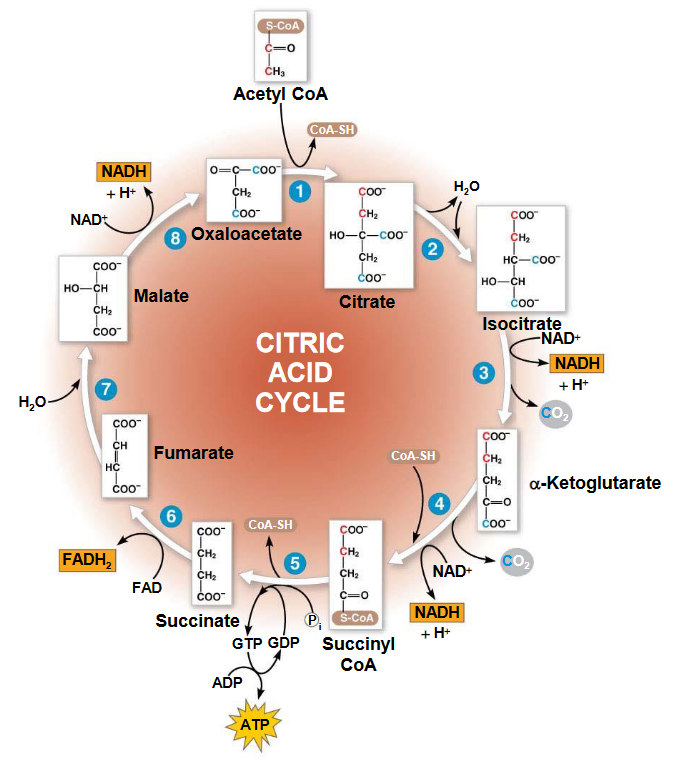
Figure 4.12: The TCA Cycle
4.3.1 Citrate formation and conversion into isocitrate
Citrate is formed from oxalatoacetate and acetyl CoA - a reaction catalyzed by citrate synthase. Thereafter, citrate is converted into isocitrate by aconitase (note that the intermediate of this conversion is cis-aconitase). This is because citrate is a tertiary alcohol and hence, cannot be oxidized.
4.3.2 NAD+ dependent isocitrate dehydrogenase
This enzyme is thought to catalyze step 3 of the TCA cycle:
Figure 4.13: Step 3 of Krebs’ Cycle
Note that the manganese ion (i.e., the Mn2+) coordinates the formed carboxyl group to polarize its charge. The green carboxyl carbon also facilitates decarboxylation by acting as an electron sink.
4.3.3 NAD+ dependent \(\alpha\)-ketoglutarate dehydrogenase
This enzyme catalyzes step 4 of the TCA cycle. The overall reaction that occurs here is mediated by an enzyme complex similar to the PDC:
- E1: \(\alpha\)-ketoglutarate dehydrogenase
- E2: dihydrolipoyl transsuccinylase
- E3: dihydrolipoyl dehydrogenase
Succinyl-CoA is a high-energy intermediate that is utilized in the next step of the TCA cycle to drive substrate-level phosphorylation of GDP to GTP in mammals (or ADP to ATP in plants and bacteria).
4.3.4 Succinate dehydrogenase
This enzyme has FAD molecules that are bound to the membrane. FAD oxidizes alkanes to alkenes and NAD+ oxidizes alcohols to aldehydes or ketones. This is because the oxidation of an alkane is sufficiently exergonic to reduce FAD to FADH2, but not enough to reduce NAD+ to NADH.
Note that FADH2 can also be reoxidized by coenzyme Q is the electron transport chain.
4.3.5 \(\Delta G^\{omicron'} and \Delta G^\omicron of TCA cycle reactions\)
Know the following graphic; the \(\Delta G^{\omicron'}\) values with boxes around them represent enzymes that are the least likely to function far under equilibrium under physiological conditions.
4.3.6 Regulatory mechanisms of the TCA cycle
The rate of ATP hydrolysis only goes as fast as the rate of ATP synthesis (which controls the rate of formation of NADH and FADH2). Hence, the oxidation of acetyl-CoA in the TCA cycle can only go as fast as electrons can enter the electron transport chain.
4.3.7 Anaplerotic pathways of the TCA cycle
Many intermediates can also enter the TCA cycle:
Figure 4.14: Anaplerotic Pathways to the TCA Cycle